David Pincus is an Assistant Professor in the Dept. of Molecular Genetics and Cell Biology. The Pincus lab is located in the Center for Physics of Evolving Systems on the 5th floor of GCIS.
The Pincus Lab studies cellular adaptation at three levels: cell biological mechanisms of adaptation to environmental stress, global principles of adaptation and resource allocation in complex environments, and the intersection of physiological stress response factors and evolutionary adaptation.
David is trained in approaches in biochemistry, biophysics, genetics, genomics, and molecular, cell, computational, systems and synthetic biology. The lab uses budding yeast and cultured human cells as experimental models.
Key project areas:
1) Quantitative cell biology of the heat shock response
2) Single-cell transcriptomics in complex stress environments
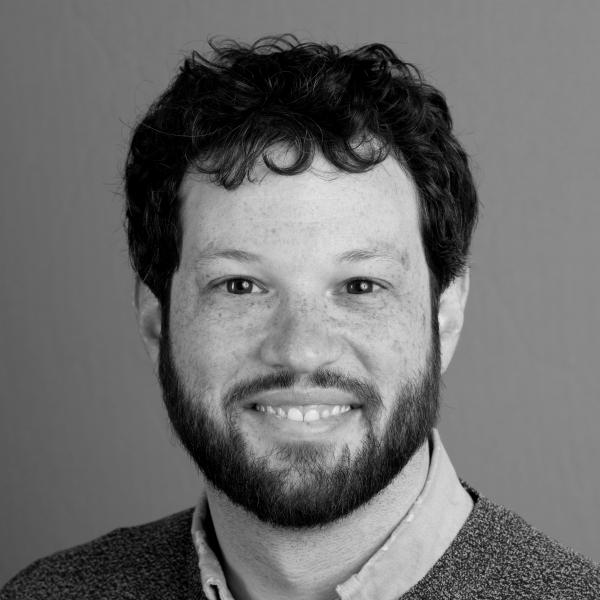
David Pincus, PhD
-
Assistant Professor of Molecular Genetics and Cell Biology
Committee on Cancer Biology
Committee on Genetics, Genomics and Systems Biology - Research and Scholarly Interests: chaperone, Hsf1, Hsp40, Hsp70, Hsp90, kinase, Phosphorylation, proteostasis
- Websites: Pincus Lab, Research Network Profile
- Contact: pincus@uchicago.edu
- Graduate Programs: Cancer Biology, Cell & Molecular Biology, Genetics, Genomics & Systems Biology